With new faculty hires over the last few years, Drexel Materials has increased its capabilities in energy and polymer research. These new additions have necessitated a look at our laboratory facilities and have resulted in the expansion of research facilities during the 2015-2016 academic year. Assistant professors Andrew Magenau and Ekaterina Pomerantseva have both moved into newly renovated space in the Bossone Research Enterprise Center. In addition, a new space has been outfitted for two donated molecular beam epitaxy (MBE) instruments for ongoing research by Professors Steven May and Jonathan Spanier.
The Macromolecular Materials Group (MMG), led by Magenau, has recently moved into an advanced polymer synthesis laboratory where they are conducting cutting-edge research on soft materials. “We have three main research thrusts focused on developing novel electrochemical polymerizations, mesoporous network structures, and supramolecular assemblies,” shares Magenau. These processes and soft materials are envisioned for high-throughput polymerizations and a range of applications spanning from solid-supported catalysis and self-healing polymers to anti-fouling and separation technologies. One particular interest of the MMG is the advancement of pretreatment membranes designed for improving water purification in hopes of helping to resolve the world’s current water crisis.

Jessica Weaver, a PhD student in the MMG, uses Gel Permeation Chromatography (GPC) to determine the molecular weights of polymers she synthesizes in the lab.
To build these next generation soft materials, the MMG specializes in synthesizing precision macromolecules and polymeric morphologies. Specifically, the MMG designs tailor-made soft materials from the bottom up, utilizing chemistry to synthesize custom macromolecular building blocks for construction of macroscopic structures. “With the development of controlled/living polymerizations and powerful catalytic reactions, we are now able to engineer macromolecules in a very systematic way, giving us the ability to create macromolecules with targeted molecular weights, complex microstructures, and precisely placed functional groups,” explains Magenau. At present, the group employs such advanced polymerization techniques, including reversible-addition-fragmentation polymerization and ring-opening metathesis polymerizations, coupled with polymer functionalizations accomplished through “click” chemistry and the Nobel prize winning Suzuki-Miyaura cross-coupling reaction.
An essential element of the new laboratory is its equipment, which facilitates rapid polymer characterization and synthesis. The lab now houses two Shimadzu size exclusion chromatography units providing critical analytics for verifying polymer microstructure. “Size exclusion chromatography enables us to determine the molecular weight, dispersity, and architecture of a vast array of hydrophilic and hydrophobic polymers commonly synthesized within our group,” says Magenau. The MMG’s lab also contains a Gamry potentiostat utilized for characterizing electro-active polymers and triggering electrochemical reactions for macromolecular synthesis. Furthermore, Magenau’s PhD students, David Howe and Jessica Weaver, have also begun carrying out their polymerizations and functionalizations in an Inert atmosphere glovebox for oxygen or moisture sensitive reactions and two newly installed chemical fume hoods.
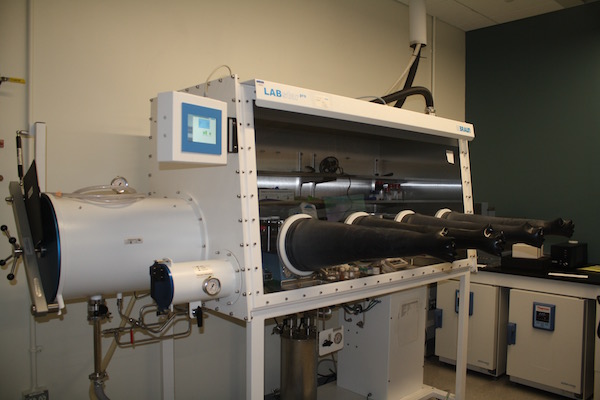
New LabStar Pro Glovebox from MBraun in the MEL. An argon atmosphere inside the box is maintained with less than 0.5 parts per million oxygen and water contamination. This glovebox is utilized to make Li-ion and Na-ion battery coin cells for electrochemical testing of different battery electrode materials. A variety of air and water sensitive solvents and lithium- and sodium-containing salts are kept inside of the glovebox so that various battery electrolytes can be created with specific, customizable compositions.
The Materials Electrochemistry Laboratory (MEL), led by Pomerantseva, researches electrochemical systems for energy storage and water treatment, which are essential to the discovery and development of new solutions for next generation devices for energy sustainability and a clean environment. Specifically, the research in MEL involves the synthesis, characterization, and electrochemical testing of new nanostructured materials. The capabilities in MEL are particularly strong for materials synthesis and electrochemical testing, including air-sensitive electrochemical systems. “We apply chemical synthesis approaches to obtain materials with a targeted crystal structure, composition, and morphology, enabling desired electrochemical properties and bridging the gap between chemistry and materials science,” says Pomerantseva.
Efforts in MEL are targeted at discovering new materials for advancing batteries, supercapacitors and water desalination systems. The lab especially focuses on chemistry and electrochemistry of materials suitable for beyond-lithium ion reversible intercalation processes. This interest is motivated by the growing demand for affordable energy storage devices and fresh water. “Our goal is to significantly improve the efficiency of beyond-lithium ion electrochemical devices”, says Pomerantseva. One of MEL’s projects explores tunnel structured manganese oxide nanowires for Na-ion battery electrodes with high energy and high power. Due to the stability of manganese oxides in aqueous solutions and one-dimensional morphology, these materials also show promise for the manufacturing of water desalination membranes with selective extraction of ions from ocean water. MEL also has an on-going project on the synthesis of a new class of layered materials with large interlayer spacing – chemically pre-intercalated vanadium oxides. “By inserting different inorganic ions through a wet chemistry synthesis approach, we can control the interlayer distance in the obtained materials and study their storage ability and ion transport through these materials’ large open interlayer diffusion channels,” shares Pomerantseva. Pre-intercalated ions also have the potential to stabilize the crystal structures of the materials and improve the cycle life of the batteries.
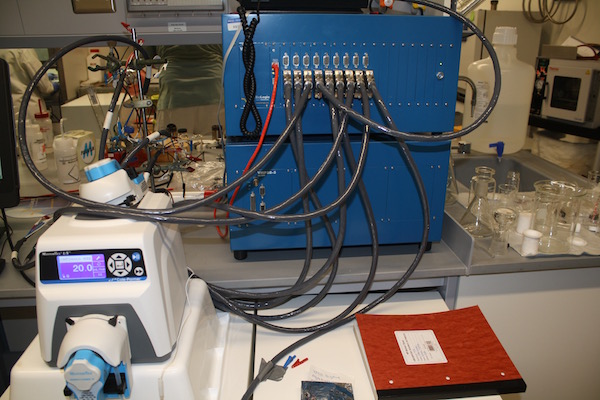
This Biologic potentionstat in the MEL is utilized for advanced electrochemical investigation of metal oxide materials for Li-ion and Na-ion batteries, pseudocapacitors, and capacitive deionization systems. Many electrochemical techniques can be performed with this machine, such as cyclic voltammetry, galvanostatic charge/discharge, electrochemical impedance spectroscopy, and chronoamperometry. The white pump with the LCD display on the left of the image is a peristaltic pump for efficiently moving salt water through a custom built capacitive deionization cell that is utilized to evaluate materials’ abilities to remove salt ions from water.
The laboratory is equipped with four ovens, operating at temperatures of up to 330oC, and multiple acid digestion vessels for hydrothermal synthesis of materials. In addition, the laboratory hosts a furnace for high-temperature materials annealing. The reduction in materials particle size and homogenization of solid materials mixtures is assisted by grinding in a planetary ball mill with agate jars and media. The laboratory is also equipped with a high precision balance, pH meter, ultrasonic bath, and multiple stirring plates with controlled temperature. To analyze the thermal stability of materials and evaluate crystallographically bound water content, which can be critical for some energy storage systems, the laboratory is equipped with a thermogravimetric analyzer.
Electrodes fabrication is performed using a slurry casting technique followed by drying in vacuum ovens. Coin cells and both two-electrode and three-electrode Swagelok cells are routinely used for electrochemical testing of battery and pseudocapacitor electrode materials. The laboratory hosts an M. Braun Labstar Pro Glovebox system with inert atmosphere for battery assembly and testing with water-sensitive electrolytes. Electrochemical testing is performed using a battery testing station with twenty channels (Arbin Instruments, BT-2000) and multi-channel potentiostat with impedance measurement capability (Biologic, VMP3). Materials for the flowable electrochemical devices, such as water desalination systems, are investigated by integrating the potentiostat with the digital peristaltic pump. The conductivity of solutions is measured using a flow-through conductivity probe. The characterization of materials for electrocatalytic applications, such as electrochemical water splitting, is carried out using a rotating disk electrode from the Pine Research Instrumentation company. MEL’s materials synthesis and electrochemistry testing equipment combined with the characterization capabilities in Drexel’s Core Facilities, in addition to collaboration with the National Laboratory User Centers, allows the group to tackle integrated water-energy problems to fulfill a mission to develop innovative materials for energy storage and water treatment.
The MBE Lab, also located in Bossone, was made possible by a donation of two MBEs from L-3 Communications, a company that specializes in aerospace, communication, and electronic systems. The two donated instruments had been previously used by L-3 and were cleaned and decontaminated prior to arriving at Drexel.
The MBEs enable researchers to grow new materials on a substrate by taking several elements, placing each distinct element in one of the MBEs ports, and heating them until they start to evaporate atoms. The atomic “beams” are directed at a substrate on which crystals of new materials can be grown layer by layer. The MBEs enable the researcher to control the flux and dosing of the beams to the surface of the substrate and allow for high quality crystal growth with atomic precision.
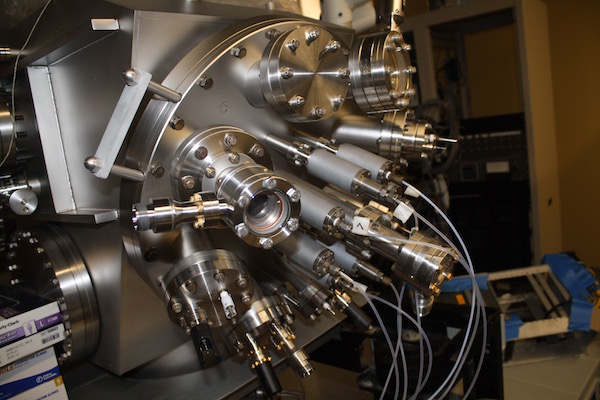
Image of ports where elemental sources are contained within the molecular beam epitaxy (MBE) system.
“By growing crystals in an MBE, we can layer atoms in ways that wouldn’t normally occur in nature, allowing us to create new materials or interfaces for important devices,” says May, whose Oxide Films and Interfaces Group will be one of the primary users of the new space. May’s group, which also has an MBE in its primary lab, grows complex oxides, which can be grown with almost all of the elements in the periodic table, enabling a wide range of physical characteristics. “Synthesizing new materials in this way allows us to hand-pick elements that have particular properties that may be favorable to one application or another,” adds May. “Some properties that may be appealing include magnetism, semiconducting behavior, or ionic conductivity, for example.” It is possible to get more functionality (properties) into a single sample by creating heterostructures, which consist of multiple distinct materials stacked together with abrupt interfaces. Semiconducting heterostructures made by MBEs are currently used in lasers, LEDs, and transistors in phones. By making oxide heterostructures, the researchers hope to realize new types of electronic or energy conversion devices.
While there are a number of different ways to manufacture new materials, MBEs are known for their high quality materials because they are clean, with the deposition occurring in a vacuum to minimize impurities. The atoms of each element are slowly evaporated by heat, rather than being bombarded, making the process chemically precise and energetically “gentle.” A major limitation that the MBE presents is that there are only a certain number of ports that an MBE has. Each port can only contain one element for evaporation, meaning the creation of a new material is limited by the number of distinct elements that can fit into an MBE.
“Having more MBEs will enhance the array of compositions that we can explore and will allow us to do more high-risk discovery of lesser explored materials,” shares May. The new MBEs also allow for a larger sample. May’s current MBE can create materials on 1 x 1 cm wafers while the newer MBEs can deposit elements on 2 or 3” wafers. This can assist in scaling up electronic device manufacturing as well.
While May will use one of the MBEs with his research group, Spanier will be in charge of the other MBE for his group, the Mesoscale Materials Laboratory. May is additionally hoping to use the MBE in an advanced undergraduate or graduate class to provide students with a hands-on educational experience focused on vacuum components and thin film deposition.