We need better ways to store and use energy, that’s no secret. Cell phones need charging every day, electric cars only have a range of about a hundred miles, and our ability to use solar and wind energy to feed the power grid is still very limited. These are things we’ve taken for granted, but if you look, historically, at the rate at which our technology improves — just think about cell phones and computers in the last 20 years — it’s easy to see that this area of technological development has severely lagged.
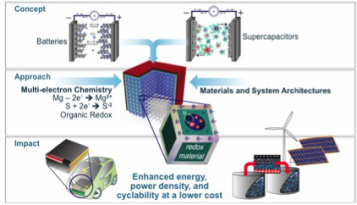
Conceptual presentation of development of fully integrated rechargeable hybrid battery-supercapacitor (supercapbattery) electrical energy storage devices. Image courtesy of K. Jost, Drexel University.
While there are a number of political, philosophical, and theoretical explanations for why energy storage development has fallen behind, experts agree that if the problem is going to be fixed in our lifetime, it needs to start now.
Energy storage is a limiting factor that researchers have been aware of for quite a while, but their work to improve our storage devices has taken many, disparate directions. In a recent edition of Nature Communications, Drexel Materials researchers Yury Gogotsi, PhD, and Maria Lukatskaya, PhD, along with Bruce Dunn, PhD of UCLA, who have been surveying the landscape of energy storage research for years, offer a unified route for bringing our energy storage and distribution capabilities level with our energy production and consumption.
Lukatskaya and Gogotsi unpacked the problem and offered up three ways in which energy storage research and development need to change right now to get things moving in the right direction:
So, the directions where we want our energy storage devices — such as batteries — to go are pretty intuitive: we want them to store more energy per unit of volume (or mass) so that it would provide longer autonomy times for portable electronics without making them bulkier. We also want to enable fast charging of the devices, so that five minutes of charging would provide full-day power for device operation. And last, but not least, we want to increase the lifespan of batteries — meaning the number of charge/discharge cycles they can undergo without performance degradation.
To achieve that, we need to rethink conventional electrode architectures and materials that are currently used in energy storage devices, such as batteries and supercapacitors.
Clean up all the wasted space
For example, in state of the art batteries, too much volume is occupied by the cell components that do not store charge. It is estimated that in smaller devices more than 80 percent of the volume is occupied by the inert cell components: current collectors, separators, and casings. So new design concepts that minimize use of current collectors would lead to substantial improvement in energy that can be stored per unit of mass or volume of the device.
Come up with a better recipe
Secondly, new electrolyte and electrode chemistries should be explored. Currently, oxide materials dominate the “insides” of batteries. Oxides have many advantages, being among the most studied material, and they have provided a reliable energy storage solution for quite a while, but in order to address growing needs for high-energy batteries, other electrode materials should be explored that have high electrical conductivity and can enable multielectron redox reactions (storing more charges per atom than lithium).
Get electrons and ions on the expressway
In order to make energy storage devices fast, it is again necessary to reconsider electrode architectures to ensure rapid accessibility of ions and electrons toward active sites. Basically, we need to create such architectures where, instead of a “maze,” ions can move on “highways” providing fast charging.
Gogotsi is Distinguished University and Trustee Chair professor in Drexel Materials and director of the A.J. Drexel Nanomaterials Institute. Lukatskaya (PhD 2015), was a doctoral candidate in the Department of Materials Science and Engineering when she worked with Gogotsi on this research. She is now a post-doctoral research fellow at Stanford University.