Controlling What Goes on 'Between the Sheets' is Key to Optimizing MXenes' Abilities
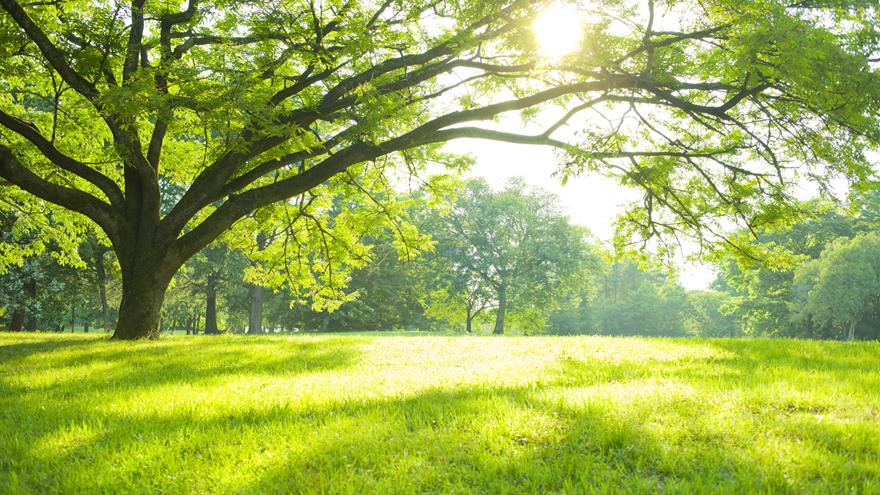
- Men and Residents of Higher Crime Areas See Greater Benefit from Community Parks, in Reduction of Deaths from Heart Disease
- Faculty Highlights: Recent Awards and Grants
- A Framework for Community-Driven Environmental Justice Guided by the Community
- Researchers Develop LLM to Identify and Suggest Alternatives to Words That Stigmatize
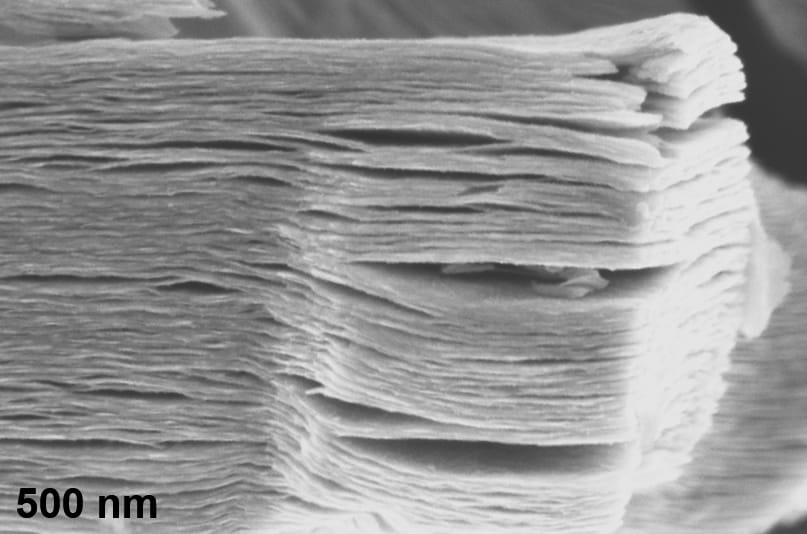
By using direct detection electron energy loss spectroscopy to examine the surface chemistry of the layers of two-dimensional MXene materials, Drexel researchers are able to better understand how to optimize them for specific uses, such as blocking electromagnetic radiation, transmitting radio waves, or detecting chemicals in the air.
Amidst a surge of research over the last two decades focusing on the special properties of structures that are just an atom or two thick, called “two-dimensional” materials, researchers at Drexel University have steadily been uncovering the exceptional properties of one family of these materials, called MXenes. The researchers now know that MXenes are highly conductive and extremely durable, they can block electromagnetic interference, sense chemicals in the air, remove salt from water, and capture hydrogen. They’ve made a strong case to be involved in the future of energy storage, wireless communication and wearable technology. But before that can happen researchers need to understand why MXenes can do what they do — and how they can be designed to do it better.
As two-dimensional materials, MXenes are largely defined by their surfaces, yet researchers are in the early stages of directly measuring how the surface chemistry of MXenes influences their performance. Researchers in the Dynamic Characterization Group in Drexel’s College of Engineering recently addressed this question in the journal Nature Communications. Their study suggests that engineering the atoms bonded to the surfaces of MXenes and the molecules between their layers could dramatically improve various properties of the materials.
In their examination of MXene surface chemistry, the researches relied on a new electron microscopy technique – developed at Drexel in 2016 – which allows unprecedented measurement of the property-defining surface chemistry in real time.
“While the idea of controlling MXene properties by changing their surface termination and intercalation has always been a key objective in advancing these materials, we are the first to directly achieve this goal and lay the groundwork for engineering these materials to improve conductivity and explore the possibility of developing semiconducting, magnetic and topologically insulating MXenes,” said Mitra Taheri, PhD, Hoeganaes professor and head of the Dynamic Characterization Group, the lead author of the study. “The holy grail is to have control over what goes on ‘between the sheets,’ so to speak. We are demonstrating a major step toward termination engineering through the use of novel in-situ TEM techniques and our direct detection spectroscopy technology.”
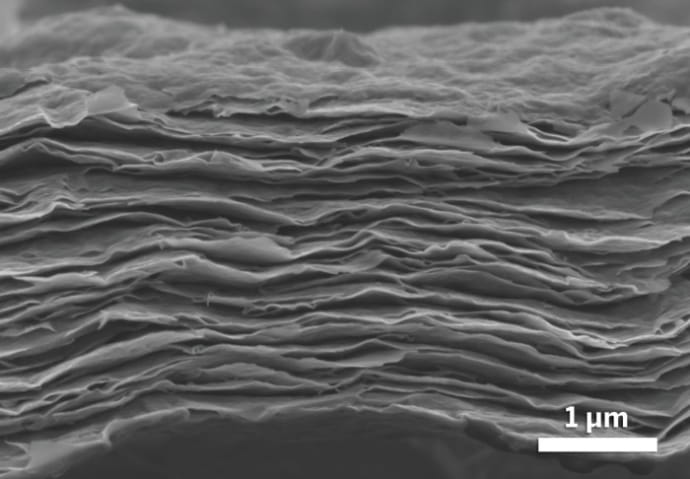
MXenes, which were first discovered at Drexel in 2011, are made by chemically etching a layered ceramic material called a MAX phase, to remove a set of chemically-related layers, leaving a stack of two-dimensional flakes. Based on the exact chemical etchant used, the atomic species left bonded with the flake surfaces – the termination species – and the molecules which get stuck in between the flakes – the intercalants – will vary. Researchers have speculated that the interplay between the MXene, termination species, and intercalation species have something to do with MXenes’ conductivity.
Now they’ve confirmed it.
Some 30 different types of MXenes have been produced at Drexel, and this study looked at the behavior of three that are frequently explored for applications. The goal of the researchers was to measure the conductivity of these materials before being tested, and then to monitor it as intercalants were removed and the surface chemistry of the flakes was altered.
To do this, the team incrementally heated the materials in a vacuum to temperatures as high as 775 degrees Celsius. During the heating process, the team monitored both the electronic resistance of the material — a way of determining its conductivity — as well as observing the chemical dissipation, or de-intercalation, of the intercalant in real time. To make these measurements, the researchers used a technique they’d previously developed — called direct detection electron energy loss spectroscopy, which is ideal for monitoring chemical changes in 2D materials.
The same process was able to monitor and study the release of the termination atoms from the surface of the MXene flakes. In both instances, measures of the electrical resistance of the material, revealed that they became more conductive as intercalants and termination species were eliminated.
“In our study, we started with MXenes that had a mix of oxygen, hydroxide, and fluorine termination species, and we showed that as you partially remove these surface termination groups, the conductivity increases significantly. This is also true as water and organic molecules are de-intercalated,” said Jamie Hart, a doctoral researcher in Department of Materials Science and Engineering and an author of the research. “Importantly, by testing these materials in the electron microscope and measuring them with electron energy loss spectroscopy, we were able to establish a causal relationship between intercalation and termination loss and improved conductivity.”
Though this confirms a theory that has been speculated upon for some time, Hart notes that it has been nearly impossible to accurately induce, track and measure the effects of these chemical changes until now. So this discovery is significant not only because it shows the source of MXenes’ behavior, but also how the behavior can be altered.
“Most experimental studies looking at MXenes are geared toward a specific application — for example, using MXene to make a battery and optimizing the fabrication and design to maximize battery performance,” Hart said. “Our study asks fundamental questions about the properties of MXenes and our findings provide clear guidelines for how to improve conductivity in MXenes, which should directly translate into improved performance for applications such as antennas and electromagnetic interference shielding.”
The findings are an important step toward optimizing MXenes for various applications — wearable electronics, energy storage and electromagnetic interference shielding, are among those on the horizon — as well as understanding how to make them stable in atmospheric conditions for extended periods of time. They also point the way toward creating magnetic MXenes that could be used for data storage devices.
“This sort of research is foundational to the development of MXenes and their eventual integration into the devices that improve our day-to-day lives,” said Kanit Hantanasirisakul, a co-author of the study and a doctoral candidate in the lab of Yury Gogotsi, PhD, Distinguished University and Bach Professor in Drexel’s College of Engineering and director of the A.J. Drexel Nanomaterials Institute. “It will be exciting to follow the progress of MXenes now that we have a better understanding of how to control their properties.”
This research was supported by the National Science Foundation, the U.S. Department of Energy and the U.S. Army Research Office. In-situ TEM studies were performed on a DENSsolutions Heating and Biasing holder. In addition to Taheri, Hart, Gogotsi and Hantanasirisakul, Steven May, PhD, associate professor; Babak Anasori, PhD, a research assistant professor; David Pinto, PhD, a post-doctoral researcher; and Andrew Lang, a doctoral candidate, all from Drexel’s College of Engineering, contributed to this research.
Read the full study here: https://doi.org/10.1038/s41467-018-08169-8
In This Article
Contact
Drexel News is produced by
University Marketing and Communications.