Addressing the Elephant in the Circuit — Finally, a Shrinkable Alternative for Capacitors
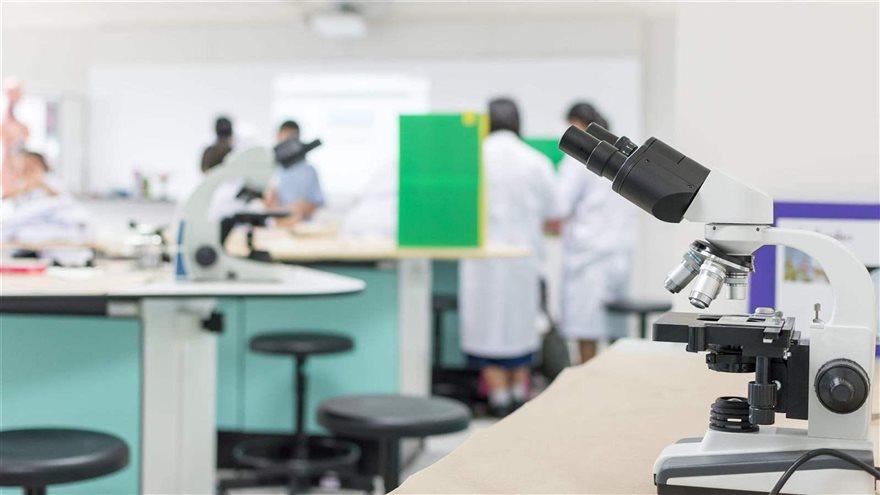
- Dragons on Fire: Kudos for Student Achievement: Fall 2024
- Children Exposed to Antiseizure Meds During Pregnancy Face Neurodevelopmental Risks, Drexel Study Finds
- Standardized Autism Screening During Pediatric Well Visits Identified More, Younger Children with High Likelihood for Autism Diagnosis
- Reporting Into the Void: Research Suggests Companies Fall Short When It Comes to Addressing Phishing
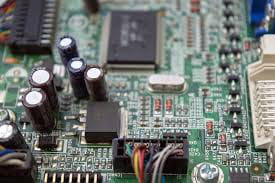
In a circuit, capacitors help to steady the flow of electrical current — absorbing surges and providing temporary power when necessary — but their size has been a limiting factor in the development of wearable technology.
One of the last remaining unshrinkable obstacles blocking the progress of fully integrated, wearable technology is the clunky component that absorbs and disburses stray electricity and converts alternating current from a power source into the direct current used by most devices. Due to a meager selection of materials that can perform those diverse functions, these components — called electrolytic capacitors — tend to be a limiting factor when it comes to downsizing electronics. But a breakthrough by materials science and engineering researchers at Drexel University and Sungkyunkwan University in Korea could eventually replace them with a capacitor so thin and flexible that it’s literally painted on.
Steady Now
The role of capacitors in electronics is to keep things steady — converting the fluctuation of an alternating current into the smooth flow of a direct current; absorbing excessive electric current or power surges that could hamper performance or damage the device; and acting as a power backup for short periods of time when there’s a disruption with the main source.
So, they’re the things that keep the buzz out of speakers and provide just enough life support to hit “save” before a laptop battery dies. Lately, they’re also being used to bridge the gap between battery and motor in electric and hybrid vehicles.
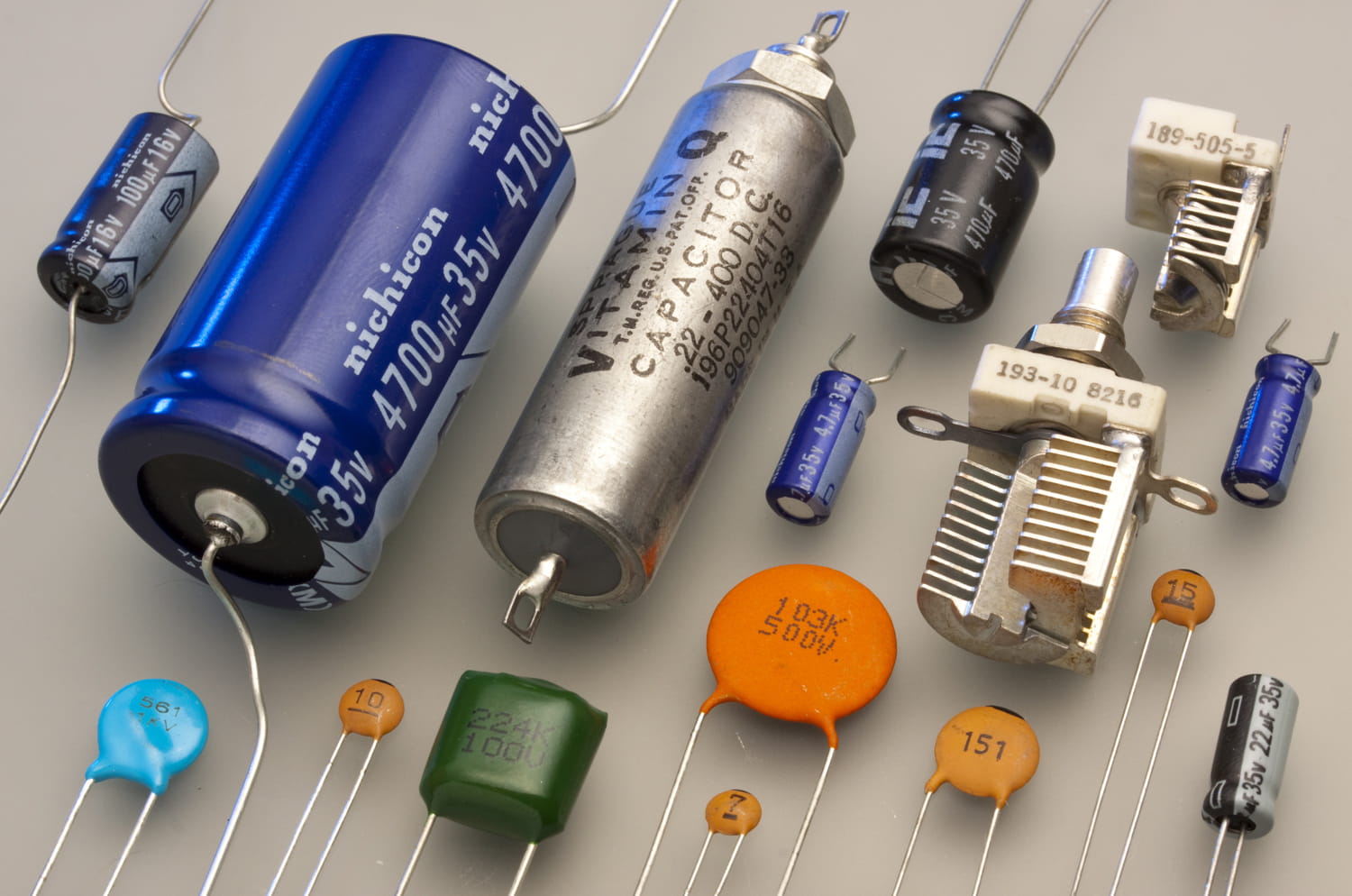
But as with most components, performance tends to diminish as engineers attempt to downsize them for use in smaller, more portable devices. The best-performing capacitors today, aluminum electrolytic capacitors, are about the size of a pencil eraser, in their smallest iteration — which is small enough for laptops and remote controls, but unwieldy for technology embedded in clothing and other wearable accessories.
Thus an advancement, like the one recently reported by Drexel College of Engineering Distinguished University and Bach professor Yury Gogotsi, PhD, and his colleagues at Sungkyunkwan in the journal Joule, could hold significant bearing for the future of ubiquitous technology because it may eliminate capacitors as a size bottleneck and also shows how the capacity and speed performance of electrochemical capacitors — or “supercapacitors” — could be greatly improved.
“The capacitance of aluminum electrolytic capacitors is typically in the milliFarads range, while our MXene supercapacitors are rated in Farads — this means their capacitance is many times larger, which would allow a much smaller device to do the same job,” Gogotsi said. “AECs are often the largest devices in personal electronics. Making them much smaller could have a big impact.”
The report suggests that capacitors made with a conductive polymer sandwiched between atom-thin sheets of a type of two-dimensional material called MXene, can absorb enough charge to power your cell phone — at least for a short time — in just a millisecond. This means it could handle quite a jolt in any system, as well as helping to keep things running when there’s a sudden loss of power.
Directing Traffic
If you think of a capacitor like a parking deck, ideally it would have lots of parking spaces and be large enough to accommodate a lot of vehicles and with a good number of well-marked entrances and exits, so it’s easy for everyone to get in quickly and exit in an orderly fashion.
At the molecular level this is what’s happening in the MXene-polymer capacitor.
The porous MXene layers provide numerous areas of ingress and egress for the moving ions of an electric current. In between the layers of the MXene “parking deck,” a conductive polymer material provides ample spaces for all the ions to park — which is why the device can accommodate an impressively high voltage and charge or discharge in a millisecond.
In addition to increasing the capacity of the device, the polymer material also imposes a sort of structure on the various layers and pores of the MXene that helps to direct traffic as ions come and go. This means the MXene-polymer supercapacitor can convert an alternating current (AC) — the rawer form of electricity that comes directly from the source of its generation — to a direct current (DC) — which is the type of electricity actually used to power devices.
Because of both its capacity and efficient management of flow, a high-performing MXene-polymer capacitor can be made much smaller than AECs.
And that’s not all.
Being Flexible
The researchers point out, as a key motivation for their work that “the rigid shape and large size of AECs are obstacles for their use in miniature and flexible electronics systems.” In order to truly “bridge the gap toward form-factor-free miniature and scalable devices” — their stated goal — the team also needed to produce a capacitor that could perform in an environment that demands flexibility.
Fortunately, the durability of materials in the MXene family have been well documented by researchers in Drexel's Department of Materials Science and Engineering over the years. MXene has proven to be one of the strongest materials of its size, but more importantly, it can maintain its properties of conductivity in a variety of forms — from a clay, to an ink, to a thin film.
The study suggests that these properties also hold true for MXene-polymer capacitors. The researchers report that they can be produced, via spray application, as a paper-thin film that maintains both is charge/discharge speed and capacity while it is bent. This means that the capacitors could be produced at any size and be part of electronics devices on nearly any surface.
This combination of speed, size and durability is unmatched by any devices currently being developed, according to the researchers.
“Breakthroughs were made to realize high-frequency ECs using nanostructured carbons for the replacement of electrolytic capacitor filters, but neither high volumetric capacitance, nor scalable device manufacturing has yet been achieved,” they write. “Large-scale flexible ECs that can provide both high volumetric capacitance and a fast frequency response are expected to resolve the current impediment for high-power applications,” the researchers write.
Gogotsi suggests the next steps for this research include improving its cycle life from the 30,000 cycles reported in the paper and continuing to optimize production techniques.
This research was funded by the Korean National Research Foundation. Read the full paper here: https://www.cell.com/joule/pdfExtended/S2542-4351(18)30508-7
Drexel News is produced by
University Marketing and Communications.