Researchers Describe the Physical Forces Underlying Sickle Cell Disease
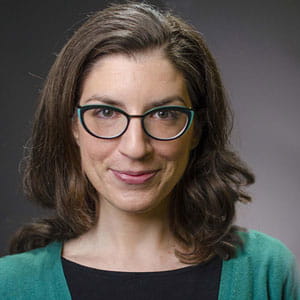
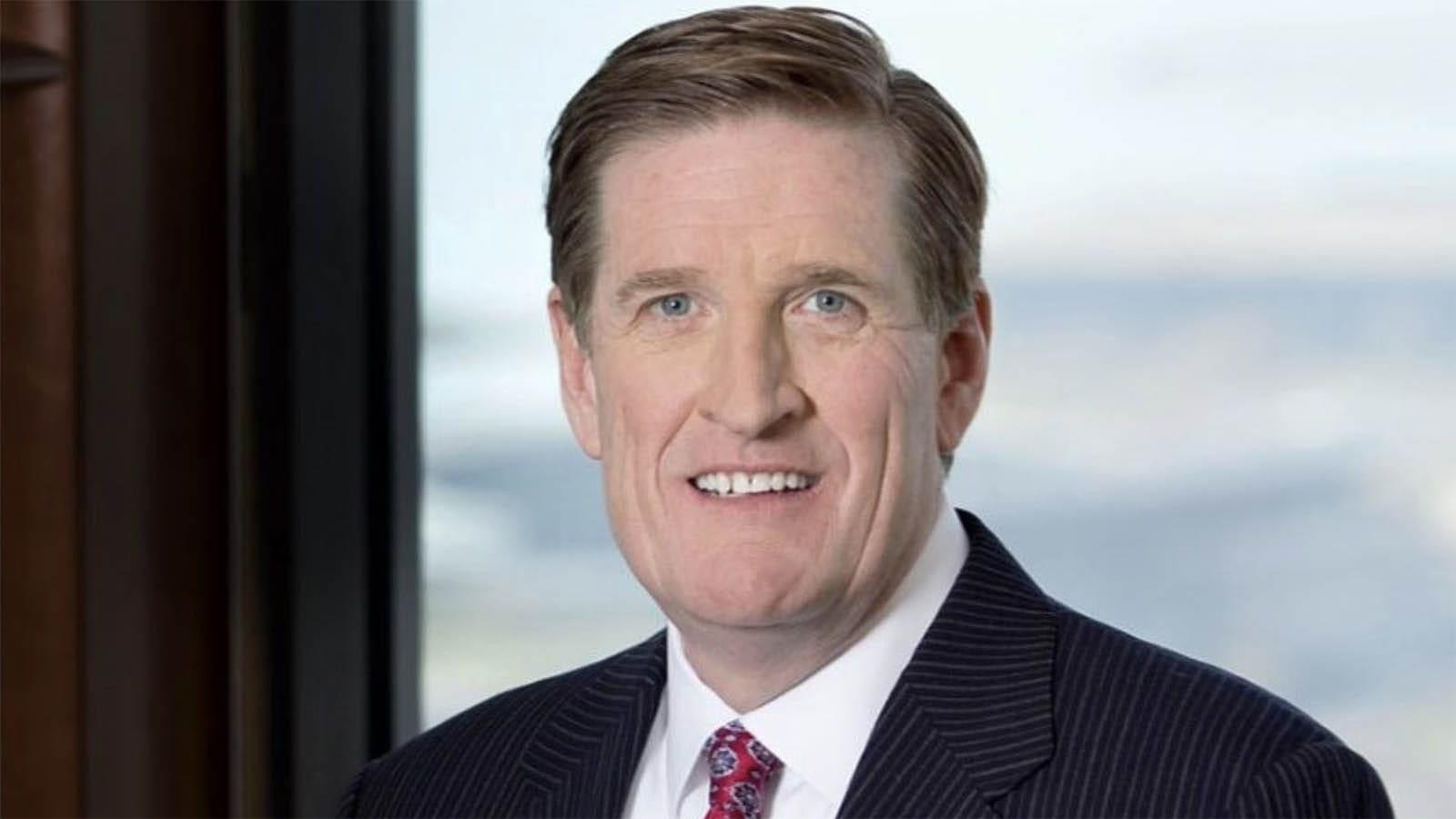
- Drexel Names Trustee and Area Business Leader Denis P. O'Brien Interim President
- Is Climate Change Keeping Patients from Vital Doctor Appointments?
- Drexel Team Identifies Drug-like Molecules That Show Early Success in Targeting Breast Cancer Brain Metastases
- Mapping the Surfaces of MXenes Atom by Atom Reveals New Potential for the 2D Materials
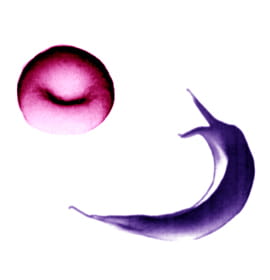
Researchers at Drexel University have identified the physical forces in red blood cells and blood vessels underlying the painful symptoms of sickle cell disease. Their experiment, the first to answer a scientific question about sickle cell disease using microfluidics engineering methods, may help future researchers better determine who is at greatest risk of harm from the disease. They report their findings in Cell Press’s Biophysical Journal today.
Capillary Blockage Conundrum
Like many scientific questions, this discovery began with a mystery. Normal, healthy red blood cells are extremely flexible, squeezing and slipping through blood vessels with ease, even passing through the smallest capillaries that are narrower than the red blood cells themselves. But in sickle cell disease, red blood cells are prone to deforming and turning rigid while flowing through the body. A seemingly logical explanation for sickle cell disease was that its symptoms – painful episodes and organ damage caused by oxygen deprivation – resulted from the rigid sickle cells forming inside narrow capillaries and then getting stuck there.
In fact, sickle cells do not get stuck inside capillaries. The symptoms of sickle cell disease come from partial obstructions in slightly wider blood vessels farther downstream—vessels wide enough that sickle cells should be wide enough to flow through. The mystery, then, was why? How do wide, rigid cells regularly pass through the narrowest channels without getting stuck?
To find out, the Drexel researchers developed an experimental setup to test flow through a model blood vessel.
“We created a channel, using microfluidic methods, that would be comparable in size to a human capillary,” explained Dr. Frank Ferrone, a professor of physics in Drexel’s College of Arts and Sciences and senior associate vice provost for Research, who was the study’s senior author.
Ferrone and colleagues took advantage of the fact that, for as long as they are carrying oxygen, red blood cells in sickle disease patients remain as squishy as healthy red blood cells. “They are the functional equivalent of a beanbag,” Ferrone said.
It is only after delivering their cargo to the body that hemoglobin molecules become prone to an internal reaction that turns the squishy “beanbag” cells rigid.
To test why the rigid cells do not get stuck in narrow capillaries, the researchers parked a red blood cell from a sickle patient at the center of their artificial narrow channel while the cell was still in its flexible state. Then, using a laser method, they induced the cell’s hemoglobin to begin the polymerization reaction that leads to sickling. Then they gradually raised the pressure at one end of the channel. They repeated this experiment multiple times with multiple different red blood cells. The amount of pressure required to dislodge the cells reached its maximum near 100 pascal.
"On the scale of pressures between arteries and veins, that's not a whole lot," Ferrone said. The pressure required to dislodge a rigid cell from inside a capillary is within the range of typical pressures in these blood vessels.
Uncooked Spaghetti in the Beanbag
Based on this experiment, Ferrone said, “we understand these processes in fundamental physical terms – we know how the stiffness of sickle cell arises, in other words – and so we have a more complete picture.”
That picture, Ferrone explained, describes what happens inside sickling cells as they turn from beanbag-like flexibility while carrying oxygen to rigid inflexibility.
That picture, Ferrone explained, describes what happens inside sickling cells as they turn from beanbag-like flexibility while carrying oxygen to rigid inflexibility.
Once the hemoglobin releases its oxygen, in sickle disease there is an ensuing molecular chain reaction between hemoglobin molecules to form long polymer chains that are rigid – a bit like uncooked spaghetti, according to Ferrone. As the spaghetti chains begin to grow inside the beanbag, the cell becomes less and less flexible.
However, if the cell is restrained inside a narrow channel when this reaction begins, its shape is physically restrained from growing outward. The rigid-spaghetti polymer chains inside the cell are blocked from growing beyond a certain length across its radial axis. They exert pressure outward on the cell membrane, thereby causing resistance. As observed in the experiment, that slight resistance requires a small amount of external pressure to dislodge cells from the capillary.
The researchers also found a relationship in their experiment that bolsters this explanation: the higher the concentration of hemoglobin in the cell, the greater the pressure required to dislodge the cell. This makes sense, Ferrone explained, because more hemoglobin would create more polymer chains pushing outward on the cell membrane.
Danger at Intermediate Speeds
Ferrone said these findings also indicate that the timing of polymerization inside sickle cells may be important to understanding patients’ susceptibility to symptoms.
The researchers described three potential scenarios for red blood cells turning rigid as they circulate in the bodies of sickle disease patients.
In the best-case scenario, the polymerization reaction is so slow that red blood cells remain flexible until they return to the lungs to pick up more oxygen.
The next-best scenario, which Ferrone said was somewhat surprising, is for hemoglobin to polymerize relatively quickly. If the cells begin to grow rigid while in a narrow capillary – the real-life equivalent of their experimental setup – then they will be forced into a skinny sausage shape. After passing through the capillary, rigid cells in that relatively thin shape can continue slipping through narrower spaces in wider vessels where partial obstructions have begun to form.
But intermediate-speed sickling is potentially most dangerous, according to the research team. If red blood cells flow past capillaries while still flexible, but later begin to grow rigid in a wider space, they are more likely to become both rigid when larger in size – making them susceptible to getting trapped in a vessel where previous sickle cells have already caused partial obstructions.
Ferrone suggested that this intermediate-speed danger may be a pitfall that investigators should avoid when developing therapies aimed at slowing the cell-sickling process.
Other authors of the paper with Ferrone were Dr. Alexey Aprelev, an assistant teaching professor of physics in Drexel’s College of Arts and Sciences, William Stephenson, a recent Drexel graduate who conducted work on this project for his undergraduate senior project in physics, Hongseok (Moses) Noh, an associate professor in the College of Engineering and Maureen Meier, a nurse at St. Christopher’s Hospital for Children.
Drexel News is produced by
University Marketing and Communications.