Dye-sensitized solar panel research
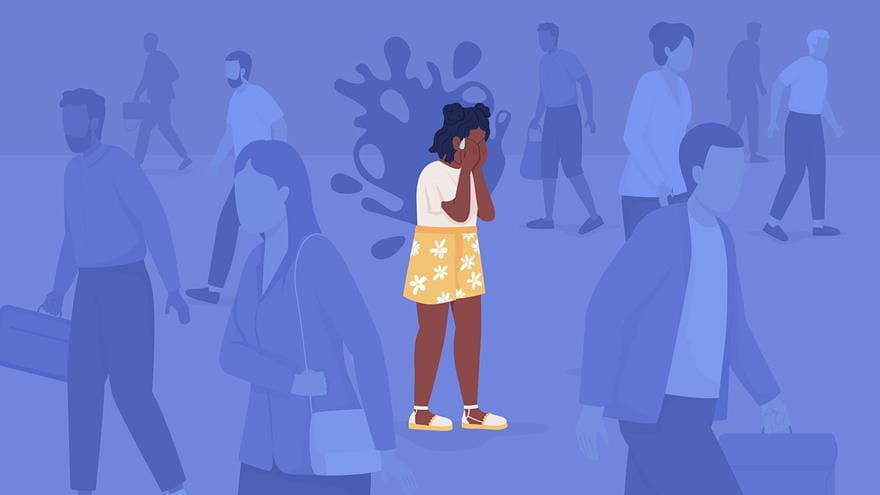
- How Do High-Profile Incidents of Racial Discrimination Affect the Eating Habits of Black Women?
- Climate Change and Sea Level Rise Pose an Acute Challenge for Cities with Combined Sewer Systems
- ‘Philadelphia Revealed,’ From the Atwater Kent Collection at Drexel University Reflects 350 Years of Philly History
- Drexel Joins EPA Effort to Prevent Greenhouse Gas Emissions from Refrigerant Chemicals
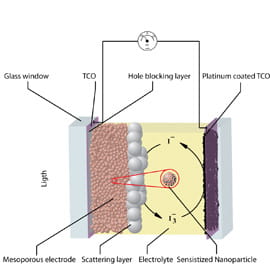
Solar panels, like those commonly perched atop house roofs or in sun-drenched fields, quietly harvesting the sun’s radiant energy, are one of the standard-bearers of the green energy movement. But could they be better – more efficient, durable and affordable? That’s what engineers from Drexel University and The University of Pennsylvania are trying to find out, with the aid of a little nanotechnology and a lot of mathematical modeling.
A three-year grant from the National Science Foundation has set the team on a track to explore ways to make new photoelectric cells more efficient, durable and affordable. The group is examining “dye-sensitized” solar panels, which capture radiation via photosensitive dye and convert it into electricity. Their goal: streamline the electron transfer process inside the solar panels to make them more efficient at converting the radiation into electricity.
Dye-sensitized solar panels currently convert about 11 to 12 percent of the sunlight that hits them into electricity. The researchers are pushing to make these panels at least as efficient as their silicon counterparts, which currently convert about twice as much radiation as the dye-sensitized panels.
Despite this relative inefficiency, dye-sensitized panels have many advantages over silicon cells. Among the advantages of dye-sensitized solar cells are low cost, ease of manufacturing and construction from stable and abundant resource materials. Also, the durability of the dye-sensitized panels, combined with their ability to absorb more sunlight per surface area than standard silicon-based solar panels, make them attractive for mainstream use. There is also the potential to make dye-sensitized cells flexible, which would open them to a variety of new applications that are not options for the more rigid silicon panels. Due to the lagging energy conversion rate of dye-sensitized cells, however, they are not as widely used as silicon panels. But with help from the group’s research, this obstacle could soon be surmounted.
“Our ultimate goal is to design and test a highly efficient dye-sensitized solar cell array through computational optimal design, synthesis and integration,” said Dr. Masoud Soroush, the project’s lead principal investigator from Drexel.
The primary strategies put forth by the group involve organizing the erratic movement of radiation-excited, or “photogenerated,” electrons into a more orderly flow and maintaining that flow through the interior of solar cell by refining the material in its electrolyte substrate.
“We are seeking the combination of electrolyte and electrode materials and cell design that provide the highest power conversion efficiency,” Soroush said. “The final design should have minimum losses in electrical conduction within the photoanode and electrolyte of the cell.”
Making a Path
At present, the process of energy collection and disbursal in a solar cell functions something like a frenetic fire drill. Solar radiation hits the photosensitive dye, which excites the electrons and sends them in an electrically charged frenzy through the field of nanoparticles comprising the electrode and finally out into the rest of the circuit.
The engineers are attempting to direct this rapid exodus of photogenerated electrons by inserting carbon nanotubes, tiny cylindrical graphite-carbon tubes that measure less than 10 nanometers in diameter, to act as corrals for their escape.
“In order for a solar cell to generate an electric current, the photogenerated electrons in the photoanode have to travel through the network of titanium dioxide nanoparticles and they encounter many boundaries between nanoparticles during the transport,” said Dr. Daeyeon Lee, the project’s principal investigator from the University of Pennsylvania. “Due to this random transit path for the electrons, a large fraction of them are lost in the nanoparticle network before they reach the indium tin oxide glass, thus failing to generate electric power.”
According to Lee, carbon nanotubes provide uninterrupted pathways for electrons, while also preventing the loss of photogenerated electrons in transit from the solar cell into the exterior circuit. With the addition of the nanotubes, the overall charge collection efficiency of the solar cell is expected to increase.
Completing the Loop
The second part of the research aims to replace the electrolyte solution that separates the electrodes inside the solar cell with a more effective polymer substance. The electrolyte serves as an internal pathway for negatively charged ions to carry electrons from the cathode (negative electrode) to the anode (positive electrode), thus completing the electrical circuit. Currently, dye-sensitized solar cells use a liquid electrolyte because it is easier for the sponge-like, porous electrode to soak up the liquid for maximum contact. It is difficult, however, to seal in the liquid, leading to leakage problems. In addition, the transit of the negatively charged species through a liquid is much less efficient than through a polymer, according to the group.
"Replacing the liquid electrolyte with a polymer will help us make a more efficient solar cell. Unlike the liquid, the polymer will not leak out of the cell and opens the door for making a flexible solar cell,” said Dr. Kenneth Lau, the project’s co-principal investigator from Drexel. “The solid polymer is also going to reduce some of the major conversion losses in the cell by closing doors that lead to electron loss that takes place with using a runny liquid.”
Lau’s group has also devised a method to get the polymer into the sponge-like electrode –a challenge which is one of the main reasons for the use of a liquid electrolyte substrate in current solar cells. “Simply put, we have invented a method for directly making the polymer inside the sponge-like electrode, rather than figuring a way to squeeze an already-made solid polymer into the electrode," Lau said.
Modeling the Cell
The variables in the research, including nanotube placement and polymer composition, could make prototype manufacturing and testing a pricey and time-consuming endeavor. But with the help of a computational material design program, developed by Soroush, the team will avail itself of rapid mathematical modeling to determine the most effective combination of materials and layout. Soroush’s program is unique to Drexel’s research in dye-sensitized solar cells and gives the team a distinct advantage in reaching its goal.
“Our predictive solid-state dye-sensitized solar cell model will allow us to establish important relations between cell performance and cell design and its material parameters,” Soroush said. “We will then use the predictive model to evaluate the cell performance over the entire cell design parameter space. By doing this we will be able to systematically search for and arrive at the design specifications that will optimize the cell’s operation.”
In This Article
Contact
Drexel News is produced by
University Marketing and Communications.