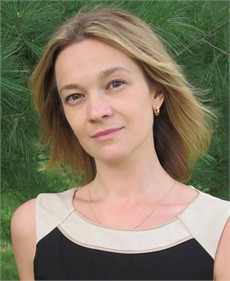
Dr. Ekaterina Pomerantseva
“Energy” was placed at the top of the list of Humanity’s Top 10 Problems for the Next 50 Years, as developed by Nobel Prize Winner Richard Smalley. This energy challenge of today requires developments in many areas including photovoltaics, catalysis, more energy efficient reactions and processes, hydrogen generation, carbon dioxide sequestration and energy storage. Assistant Professor Ekaterina Pomerantseva and her students in the Materials Electrochemistry Group (MEG) work on the development and characterization of novel nanostructured materials, systems and architectures for batteries, supercapacitors and fuel cells in order to understand electrochemical energy storage and conversion at the nanoscale. Pomerantseva’s research has two main goals: the development of new nanomaterials, architectures and systems will address fundamental electrochemical issues; and by answering fundamental questions, will tackle practical challenges and aid in the development of new nanostructured electrochemical systems for efficient superior next-generation energy storage technology.
One major research thrust of the MEG is the development of “beyond lithium” nanostructured energy storage systems. To date, lithium-ion batteries are the most successful commercially used solution for energy storage devices due to their high energy density. During lithium-ion battery operation (Figure 1a), upon discharge, lithium ions transfer from anode into cathode, a process called intercalation, through a lithium-conducting electrolyte, which is assisted by electrons transport through the external circuit. These electrons are used to power various systems. While charging, the processes are reversed, and the lithium-ion battery can be used again and again. However, the limited supply of lithium has promoted an interest in energy storage “beyond lithium,” for example, sodium-ion and magnesium-ion batteries. At the same time, it would be advantageous to use the same intercalation-based technology to create more powerful batteries.

Intercalation-based energy storage systems:
(a) schematic representation of the operation of lithium-ion batteries;
(b) schematic representation of the fragments of crystal structures of tunnel manganese oxides stabilized by potassium, sodium and magnesium;
(c) SEM images of the α-MnO2 nanowires;
(d) TEM images of the single α-MnO2 nanowire. Structural tunnels are parallel to the nanowire growth direction (c direction);
(e) galvanostatic discharge/charge curves of the cells with original and acid-leached α-MnO2 nanowires at the 2nd cycle in the voltage range of 1.5 – 4.2 V at a 0.1C current rate.
In intercalation reactions, guest ion species enter vacant space in the host material crystal structure without changing the structure significantly. Materials with tunnel and layered crystal structures favor intercalation reactions. In these cases, the guest species get inserted between structural layers or in the inner tunnel space. Manganese oxides with tunnel crystal structures are a family of materials ideal for use as electrodes in these batteries because of their high theoretical capacity, natural abundance, and environmental friendliness. (See Figure 1b for examples of the crystal structure of these materials.) These materials are built by a framework composed of MnO6 octahedra, sharing corners and edges. Different combinations of octahedral stacking result in the formation of tunnels of various sizes. Tunnels of large size are of particular interest with respect to ions intercalation, since this allows for the accommodation of ions of various radii and their transfer inside the tunnel. Potassium, sodium and magnesium ions (Figure 1b) are called stabilizing ions. They are located in the structural tunnels and can be used for charge storage. All these phases can be synthesized in the form of monocrystalline nanowires using a hydrothermal synthesis technique. Figures 1c and 1d demonstrate examples of the SEM and TEM images of the nanowires synthesized in the MEG. Hydrothermal treatment of precursors produced nanowires 20 – 200 nm thick and tens of microns long. Interestingly, one-dimensional structural tunnels in the nanowires run along the nanowire growth direction (Figure 1d).
These materials can be acid leached to modify their properties. The acid leaching of manganese oxides results in the partial extraction of the stabilizing ions and incorporation of protons into the crystal structure of the material. Acid leaching can lead to the modification of material composition, morphology, electronic conductivity and surface properties. Although all these properties are crucial for electrochemical intercalation-based energy storage, the systematic characterization of acid-leached tunnel manganese oxides has not been performed. The MEG is addressing this issue by demonstrating the detailed characterization of manganese oxide nanowires and their acid-leached counterpart. For example, original α-MnO2 nanowires showed an initial discharge specific capacity of 96 mAh/g, while the acid-leached material exhibited almost twice as high capacity values. This work forms the basis for future study aimed at an understanding of the correlation between the crystal structure, composition and morphology of the “host” matrix and nature of the “guest” ions for “beyond lithium” electrochemical energy storage.
Other research areas which Pomerantseva explores with her research group include 3D microbattery architectures, metal-oxygen batteries, pseudocapacitors and electrochemically correlated mechanical properties of thin film batteries. She also hopes to build full energy storage devices to investigate their system properties.
Pomerantseva’s ultimate goal is to make batteries that last longer and can be charged faster without increasing the volume and mass in comparison to the kinds of batteries we have today. With a focus on the next generation of energy storage, Pomerantseva and the MEG are poised to improve energy efficiency in devices of the future.